Alto™ leverages digital microfluidics technology to streamline SPR workflows by automating and miniaturizing kinetics assays.
Not too long ago, the idea of digitizing lab experiments like we digitized computing would have been unthinkable. The advent of digital microfluidics (DMF), an automated and programmable fluid-handling technology, has made this possible by enabling the programming of discrete sample droplets.
In recent years, there has been a notable surge in the development of miniaturized microfluidic devices, with the aim of consolidating entire analytical assays into a single chip-like platform to streamline and multiplex laborious conventional laboratory processes. Owing to its myriad advantages, including minimizing sample usage, reducing hands-on time, increasing instrument reliability, and eliminating user-to-user variation, DMF has been used to revolutionize processes across many industries.
At Nicoya®, we saw an opportunity to solve the challenges of label-free technology through integration with DMF, and developed Alto™, our digital surface plasmon resonance (SPR) platform. Continue reading below to learn how DMF and SPR come together to enhance productivity in the lab for biotherapeutics characterization.
Table of contents
1. What is digital microfluidics?
Digital microfluidics (DMF) is an innovative technology at the intersection of microfluidics and electronics, revolutionizing the way fluids are controlled on a miniature scale. DMF leverages an array of individually controlled electrodes to manipulate droplets like discrete bits of data, enabling the execution of programmed protocols on precise quantities of liquid on a micro- and nanoscale.
The droplets act as programmable units that can be moved, merged, split, and mixed, streamlining workflows, minimizing hands-on time and eliminating user-to-user variation.
Technology fundamentals
Electrowetting, or the modulation of wettability through voltage, provides the basis of DMF technology. Aqueous droplets tend to form beads on hydrophobic surfaces, but can be spread through the application of voltage by modulating the contact angle (Figure 1). This phenomenon was first explained by the Young-Lippman equation, where the change in contact angle, or the degree of wetting, is defined by the applied voltage (Figure 2). Researchers and innovators, including within Nicoya®, have since proposed revised forms of the equation for a more accurate characterization of droplet behavior.
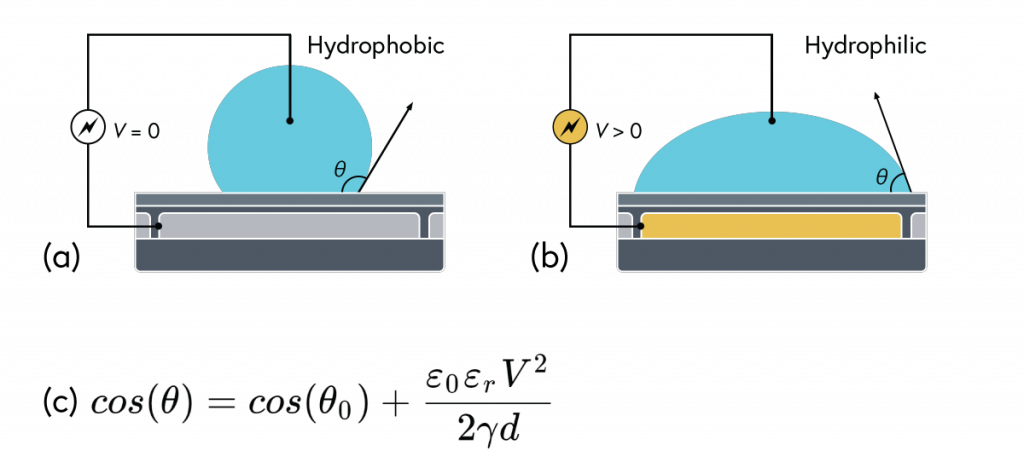
DMF devices leverage this simple concept through the intelligent stacking of multiple layers to form a simple circuit (Figure 3). The anatomy of a closed DMF system consists of two parallel plates. The bottom plate contains a substrate, a patterned array of individually controllable electrodes, a dielectric insulator to increase capacitance, and a hydrophobic layer to decrease the wettability of the surface. The top plate consists of a hydrophobic layer and a ground electrode.
The sample droplet forms the building block of a DMF-enabled assay. The sample droplet and associated buffers are sandwiched between the plates and subjected to an electric field, while the electrode, capacitive dielectric layer, and droplet form the total impedance of the circuit. Droplets are then moved by activating the voltage supply to electrodes adjacent to the droplet and simultaneously deactivating the electrode under the droplet. This manipulation of fluid enables automation of advanced fluidic protocols through the movement, mixing, and splitting of droplets, allowing you to carry out an endless array of bioassays.
When they’re not busy building cool products, our R&D team loves to get creative in the lab with DMF technology (Figure 4).
Inception and progression of technology
The concept of ‘electrowetting’ was first introduced by Lippman in 18751 and coined nearly a century later by Beni and Hackwood.2 The development of the technology towards DMF and practical applications over the next decades involved contributions from multiple researchers and institutions, with notable developments described below (Figure 5):
- Introduction of dielectrics: Berge’s 1993 paper introduced dielectrics to the technology, a material that enhanced control and stability of droplet manipulation due to its high electrical resistance and ability to store electric charge.3 While many techniques have been developed to date for droplet manipulation, electrowetting-on-dielectric (EWOD) presents the highest versatility and reconfigurability for micro- and nanolitre-sized droplet actuation.4
- Integration with microfluidics: In the late 20th century, the development of microfluidic systems enabled electrowetting to gain traction for practical applications. The integration of the two technologies was first demonstrated with mercury droplets in water5 and later with water in air6 and water in oil.7 A few years later, researchers successfully manipulated droplets on a 2D surface.8,9
- Digitization of technology: The digitization of EWOD-based microfluidics, where droplets are discretized and programmably manipulated, was described as “Digital Microfluidic Circuits” by Kim10,11 or “Digital Microfluidics” by Pollack12 and later demonstrated by Cho, Moon, and Kim in their seminal paper covering the four types of droplet manipulation they deemed necessary for digital microfluidics circuits.13 Since then, companies have taken a leap to integrate DMF with existing technologies to offer solutions with increased flexibility, reconfigurability and scalability. Notable examples include:
- NeoPrep™ by Illumina, a platform designed to provide hassle-free next-gen sequencing library prep with automation and low input. It has since been dropped from their lineup;
- ePlex® by GenMark Diagnostics, a sample-to-answer solution that streamlines the diagnostic workflow;
- FINDER™ and SEEKER™ by Baebies, platforms for point-of-care newborn screening that operate on small sample volumes and provide quick results;
- VolTRAX by Oxford Nanopore Technologies, an automated end-to-end library prep platform for DNA and RNA samples for nanopore analyses.
- Integration with SPR: We recognized an opportunity to integrate DMF with SPR, thereby leveraging the advantages of DMF and addressing the shortcomings of conventional SPR systems. Our digital SPR platform Alto™ was launched in 2020.
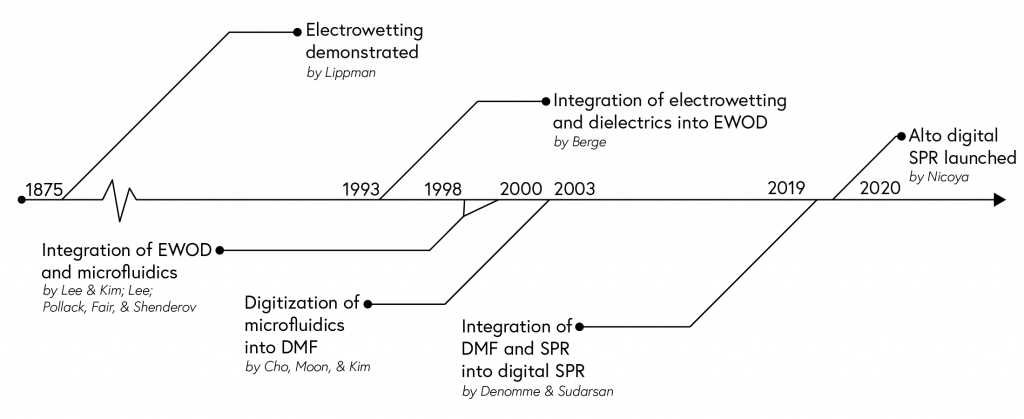
DMF has revolutionized processes across industries with its ability to miniaturize, automate, reconfigure, scale, and streamline complex operations. The past two decades have witnessed a surge in DMF research (Figure 6) and increasing applications in numerous technologies and commercial products.
2. Nicoya Alto™: DMF x Surface Plasmon Resonance
Alto™ is the world’s first and only SPR platform to integrate DMF with nanotechnology-based biosensors, providing a revolutionary solution by miniaturizing and automating assays. Designed with user-friendliness and accessibility in mind, Alto™ takes the complexity out of SPR while providing high-quality analytical data (Figure 7).
By integrating DMF and SPR, Alto™ gives you precise control over discrete sample droplets, executing a predefined protocol by automatically dispensing, transporting, splitting, mixing, fusing, and serially diluting samples prior to analysis (Figures 8 and 9).
Figure 8: Sample manipulation types of Alto™ digital SPR illustrated with coloured droplets. (a) Blue droplets are dispensed from the loading well in volumes of ~300 nL; (b) Different samples are moved around the cartridge; (c) Droplets of size ≧ 2 DU can be split into ≧ 1 DU; (d) Blue droplets are mixed to induce good transport conditions and oscillated so molecules can reach the surface; (e) Automated sample mixing, here seen with the blue and yellow droplets combining to make a green droplet and a red and yellow droplet combining to make an orange droplet; (f) An advanced feature of DMF systems, stock samples (red) can be serially diluted with buffers (blue).
How DMF streamlines SPR
More specifically, DMF improves on conventional SPR systems by:
- Reducing the impact of depletion and accumulation: With 30X less surface area than conventional SPR platforms, rapid mixing rates, and multiple droplet cycles, Alto™ is engineered to reduce the impact of accumulation and depletion.
- Decoupling interaction time from sample volume: With its DMF-powered droplet oscillation (Figure 8d), Alto™ is designed to enable unlimited sample interaction time without any sample dispersion. This allows users to optimize ligand-analyte interaction without being limited by flow rate or sample volume, increasing assay flexibility.
- Reducing signal artifacts: DMF-based delivery of samples to sensors makes it uniquely impervious to injection artifacts, thereby removing the need for the traditional buffer blank subtraction. Alto™ instead uses a detrending algorithm to correct for any baseline drift in its data. This allows users to accurately measure tight off-rates without the need for them to add lengthy buffer blanks to their experiments. Read our whitepaper to learn more.
- Reducing mass transport limitations: Alto™ is designed to minimize mass transport limitation (MTL) effects in SPR data by optimizing droplet oscillation to reduce the diffusion boundary layer to be equivalent to fast flow rates and confining the detection range to use lower amounts of ligand. Learn more in this whitepaper on MTL Kinetics Analysis with Alto™ Digital SPR.
- Improving precision and accuracy: DMF-based cartridges that automate sample manipulation allow Alto™ users to minimize hands-on and sample preparation times, decrease user-to-user variation, and improve reproducibility. Experiments testing Alto’s automated fluid handling reproducibility against mechanical pipettes and liquid handlers demonstrated superior precision and accuracy for Alto-made samples. Read our technical note to learn more.
- Increasing assay flexibility, scalability and reconfigurability: The division of fluid into discrete, independently programmable units for manipulation results in a modular and hierarchical system that can be reorganized, either through hardware or software, to provide new functionality on demand or scaled based on experimental requirements.
- Enabling a pump-free platform: DMF replaces the need for pumps and valves used in traditional fluidics-based platforms with a disposable cartridge, reducing the probability of contamination and eliminating fluidic maintenance. This makes a significant difference in the ongoing cost of operating SPR as there is rarely a need for downtime and servicing due to maintenance.
In addition, Alto™ is:
- Low footprint: The 2.2 cubic feet profile easily fits on a benchtop, so experiments can be conducted from the comfort of your own lab.
- Cost-effective: Unlike conventional SPR platforms that rely on a system of pumps and valves prone to clogging, Alto’s fluidics-free operation significantly minimizes maintenance costs and lab downtime, making it much more cost-effective than its conventional counterparts.
- Sample-friendly: Full kinetics can be obtained from 2 µL sample volumes, representing a reduction of up to 200X compared to conventional systems, including from crude and complex samples.
- High-throughput: Alto’s 16 channel design unlocks industry-leading throughput, enabling the simultaneous analysis of 64 unique samples and up to 256 interactions, with 24+ hours of unattended run time.
- Intuitive: Alto’s seamless user interface and automation provide a sample-in/answer-out solution to give you back control of your time.
- Future proof: Scale effortlessly with cloud-first software and expanded protocols unconstrained by hardware.
Comparison to conventional SPR
While surface plasmon resonance is a powerful technique for characterizing biomolecular interactions in real time, its widespread adoption is often hindered by high upfront and maintenance costs, a steep learning curve, and high sample consumption. Our technology leverages LSPR and DMF to address these barriers to access by creating a user- and sample-friendly device.
To demonstrate how Alto™ compares to conventional SPR platforms, we conducted a side-by-side experiment selecting antibodies from Human Combinatorial Antibody Libraries (HuCAL), with results summarized in this application note. Alto™ demonstrated equivalent accuracy for obtaining kinetic measurements for kon, koff, KD, Rmax with a fraction of the sample requirements (Table 1) and hands-on time (Figure 10). Our platforms have been designed with the user in mind, shortening the learning curve by automating processes and integrating an intuitive software that provides seamless user experience from assay design to data analysis.
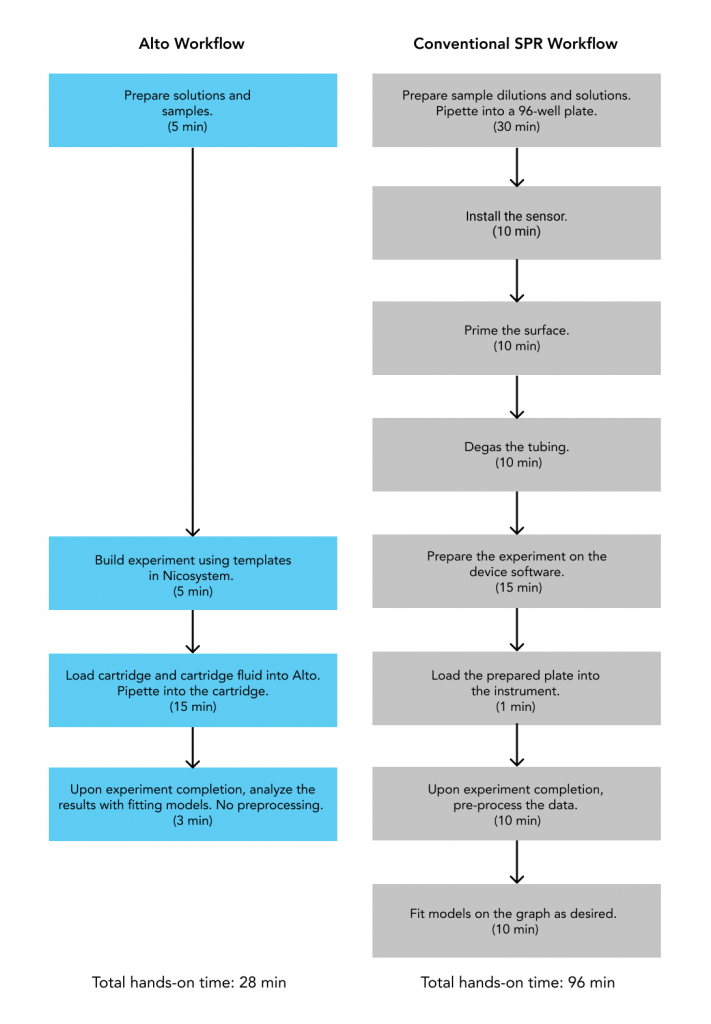
Table 1 provides a quick comparison between conventional SPR and our next-gen platform Alto™.
Table 1: Comparison between conventional SPR platforms and Alto™.
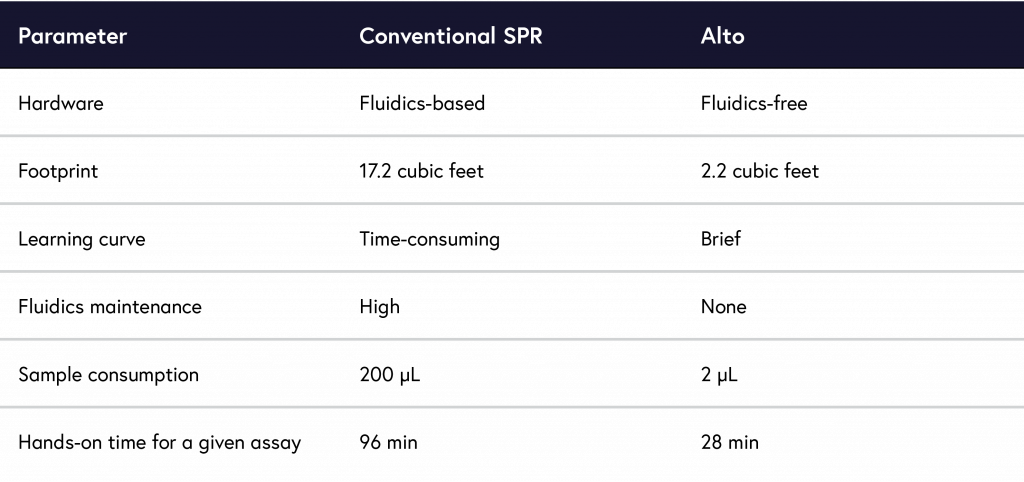
Read our blog post to learn more about how Alto™ compares to conventional SPR.
Applications and use cases
Alto™ has a wide range of applications for characterizing biomolecular interactions, including in-depth kinetics and affinity characterization, screening, quantitation, and epitope mapping and binning. It can be leveraged for characterizing a wide range of targets, including:
- Oligonucleotides: Owing to its high selectivity and specificity, Alto™ is well suited to characterize the reverse complementary binding of oligonucleotides. When put to the test, Alto™ generated accurate kinetics and affinity data with high repeatability and comparability to conventional SPR platform, with results summarized in this app note.
- Fcγ receptors: By streamlining the experimental workflow and removing the need for labeling molecules, Alto™ is perfect for characterizing the binding kinetics of antibody subclasses to Fc gamma receptors. In an experiment using mouse FcγRI and IgG2A, Alto provided data on par with a BLI instrument without significant contributions from mass transport. More detail can be found in this app note.
- Monoclonal antibodies: Given its high selectivity and specificity, ability to characterize MoAs, and suitability for large molecules, Alto™ is a good fit for therapeutic monoclonal antibody characterization. We ran experiments using IL-6 and tocilizumab, with Alto™ generating accurate data and analyzing up to 48 data points in a single cartridge. Results are summarized in this app note.
- Biosimilars: By streamlining ligand binding assays (LBAs), Alto™ brings efficiency to biosimilar development, presenting important implications for cost-savings, accelerating time to market, and increasing accessibility. Using PlantForm’s vivoXPRESS®-derived pembrolizumab, we conducted proof-of-concept LBAs, with results summarized in this app note.
- Bispecifics: With its ability to characterize sequential binding events within a single assay, Alto™ is uniquely suited for biospecifics research. Learn how we helped power Immunocore’s ImmTAC® bispecific development by reading our app note.
- Epitope diversity: By collapsing 16×16 epitope binning runs into pre-designed, automated assays, Alto™ reduces the cost and complexity of antibody discovery. We teamed up with Sino Biological to demonstrate Alto’s enhanced binning feature against their Influenza A hemagglutinin (HA), with results presented in our app note.
- Viral targets: Alto’s ability to simultaneously characterize multiple monoclonal antibodies against multiple viral proteins was leveraged by a team of researchers led by Emory University’s Matthew Woodruff. Their findings, presented in a Nature study, shined a light on the origins, breadth, and resolution of autoreactivity in severe COVID-19. Read our blog post to learn more.
As research and development in this field continues to advance, DMF is poised to transform various industries by enabling precise fluid manipulation on a microscale. Its integration with SPR has taken the technology to the next level, circumventing limitations of conventional SPR and introducing advantages of digital automation. And Alto™ is leading the way. With its ability to execute complex protocols on a miniature scale, digital SPR holds great promise for applications in early drug discovery, high-throughput screening, point-of-care diagnostics, and lab-on-a-chip devices.
Interested in learning more about Alto™ Digital SPR? Book your demo today!
References
- Lippmann G. Relation entre les phénomènes électriques et capillaires. Ann Chim Phys. 1875;5:494.
- Beni G, Hackwood S. Electro‐wetting displays. Appl Phys Lett. 1981;38(4):207-209. doi:10.1063/1.92322
- Berge B. Électrocapillarité et mouillage de films isolants par l’eau. C R Acad Sci. 1993;317(2):157-163.
- Barman SR, Khan I, Chatterjee S, et al. Electrowetting-on-dielectric (EWOD): Current perspectives and applications in ensuring food safety. J Food Drug Anal. 2020;28(4):595-621. doi: 10.38212/2224-6614.1239
- Lee J, Kim CJ. Liquid Micromotor Driven by Continuous Electrowetting. Proceedings of IEEE Micro Electro Mechanical Systems Workshop. January 1998;538-543. Heidelberg, Germany. doi: 10.1109/MEMSYS.1998.659815
- Lee J. Microactuation by Continuous Electrowetting and Electrowetting: Theory, Fabrication, and Demonstration. PhD Thesis. University of California, Los Angeles; 2000. Accessed June 14, 2023.
- Pollack MG, Fair RB, Shenderov AD. Electrowetting-based actuation of liquid droplets for microfluidic applications. Appl Phys Lett. 2000;77:1725-1726. doi: 10.1063/1.1308534
- Fan SK, de Guzman PP, Kim CJ. EWOD Driving of Droplet on NxM Grid Using Single-Layer Electrode Patterns. Tech. Dig., Solid-State Sensor, Actuator, and Microsystems Workshop. June 2002;134-137. Hilton Head Island, SC.
- Gong J, Kim CJ. Two-Dimensional Digital Microfluidic System by Multi-Layer Printed Circuit Board. Proceedings of IEEE Conference MEMS. January 2005;726-729. Orlando, FL. doi: https://doi.org/10.1109/MEMSYS.2005.1454032
- Kim CJ. Integrated Digital Microfluidic Circuits Operated by Electrowetting-on-Dielectrics (EWOD) Principle. Granted in 2000 by Defense Advanced Research Projects Agency (DARPA), award number N66001-0130-3664
- Kim CJ. Micropumping by Electrowetting. Proceedings of the ASME International Mechanical Engineering Congress and Exposition. November 2001. New York, NY.
- Pollack MG. Electrowetting-Based Microactuation of Droplets For Digital Microfluidics. PhD Thesis. Duke University; 2001. Accessed June 14, 2023. http://microfluidics.ee.duke.edu/doc/Pollack.thesis.pdf
- Cho SK, Moon H, Kim CJ. Creating, transporting, cutting, and merging liquid droplets by electrowetting-based actuation for digital microfluidic circuits. J Microelectromech Syst. 2003;12(1):70-80. doi: 10.1109/jmems.2002.807467